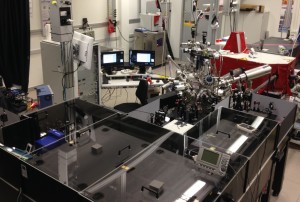
Researchers at ETH Zurich are using second harmonic generation (SHG) effect as a probe to follow the spontaneous polarization alignment of epitaxial ferroelectric films in real time, as they are being created by pulsed laser deposition. This technique provides unique insights into the factors influencing the polarization of technology relevant oxide ultrathin layers, with potential benefits for the design of faster, nanoscale electronic components with improved functionality for memory devices, RFID, and display applications.
Ferroelectrics
Ferroelectrics are a class of materials that are already commonly used in the electronics industry to form capacitors and are used in extremely thin layers in integrated circuits to form storage bits in some types of RAM and in RFID cards. These “oxide” applications depend on the fact that ferroelectric materials can be electrically polarized and thus possess a permanent electric dipole. Moreover, the direction of the dipole can be controlled by the application of an external electric field. Ferroelectrics are analogous to ferromagnetic materials, which is how they got their name in the first place. And like ferromagnets, the ferroelectric materials may possess domains characterized by differently oriented polarization states.
Ferroelectric materials have tremendous potential in ultrathin heterostructures for low energy consuming electronic components, with atomic level control of design and fabrication. Moreover, the use of nanometer-thick ferroelectrics delivers thinner, smaller capacitors that require lower operating voltages congruent with the relentless drive in integrated electronics for smaller, denser circuits with higher functionality. However, much of the fundamental physics associated with their key properties (polarity, polarizability, strain, etc.) is not sufficiently understood for thin layers of just a few unit cells in thickness, and this limits their implementation in integrated circuits. For instance, below a certain minimum thickness - called critical thickness - these materials have no intrinsic polarization. This thickness is a material-specific function that also varies according to the strain in the layers and the environment in which the film is grown (charge screening electrode, growth atmosphere, etc.). Existing technologies do not have the sensitivity and selectivity to study the polarization and other electronic properties of these films within thin heterostructures. A team of researchers at ETH Zurich led by Dr. Morgan Trassin and Prof. Manfred Fiebig, developed an non-invasive optical method powered by an ultrafast amplifier/tunable OPA combination (Coherent Astrella/TOPAS) that has successfully measured key properties in model ferroelectric (BaTiO3) and multiferroic (BiFeO3) ultrathin films, both during the actual deposition process and even after overlaying with another material. (Multiferroics are materials that possess more than one ferro-type property, e.g., ferroelectricity and ferromagnetism). Now they are using this powerful method to study films as they are created and then incorporated within heterostructures in the form of actual model devices.
SHG as a polarization probe
When laser light interacts with materials featuring symmetry breaking and long range order, a small amount of the light is converted to the second harmonic, i.e., light at twice the frequency, or half the wavelength. This second harmonic generation (SHG) effect is widely used in lasers for example to convert infrared to visible light and visible light to ultraviolet. Here the beam passes through millimeters of a material such as lithium niobate with a very high non-linear coefficient (χ) and as much as 50% of the incoming beam can be frequency doubled. In microscopy for cell biology applications, weak SHG signals are generated by collagen fibers which allows them to be selectively imaged by using dichroic filters to separate the small SHG intensity from the much more intense scattered laser light. Within material sciences, SHG has already been used in the past with thicker ferroelectric samples, and has been proposed as a method to investigate ultrathin layers of ferroelectric oxide material used in electronics, thanks to the advantages of being a non-destructive technique with a large working distance. However, before the ongoing work by the ETH researchers described here, no-one had previously demonstrated in situ SHG (ISHG) i.e., during the ferroelectric film growth process.
Dr. Trassin explains, “We wanted to see if we could use ISHG and obtain sufficient signal-to-noise based on films ranging from zero thickness to a few unit cells. Since the SHG effect is based on the intensity of the transient electric field and its interaction with polarizable electrons in the target material, it is highly sensitive to polarization. So we reasoned we could use the polarization dependence of SHG to map the alignment of domains in a ferroelectric film. And more importantly we wanted to investigate the potential of ISHG to follow the polarization (and depolarizing field related effects) of ultrathin films, during the epitaxial deposition process.” They chose pulsed laser deposition (PLD) as the method of choice to create the films, since the deposition rate is deterministic and is set by the pulse rate of the laser and other controllable factors. ISHG is however expected to be compatible to any growth process environment. Figure 1 schematically illustrates their setup.
Key elements of the setup used by the ETH team to monitor film polarization in situ during the deposition process.
Combining SHG and PLD in real time
The Trassin team designed a vacuum deposition chamber for this purpose - see figure 2. They began with BiFeO3 films grown epitaxially on SrRuO3-buffered (001)-oriented SrTiO3 (BFO|SRO|STO) at temperatures up to 700 °C. The pulsed laser deposition is driven by a krypton fluoride excimer laser (Coherent LPX) operating at 8 Hz. The film thickness is monitored throughout the PLD process by using the well-established quantitative method of reflection high energy electron diffraction (RHEED).
To implement SHG, it is important to minimize any non-linear optical signals due to bandgap excitation of the substrate and film. The ETH researchers accomplish this by using a laser excitation wavelength that is well separated from these resonances. The SHG signal intensity is determined by the (peak power)2 of the laser source, so to maximize the expected weak ISHG signals they decided to use the high energy output pulses from an ultrafast amplifier (Coherent Astrella) and tunable optical parametric amplifier (OPA accessory (Coherent TOPAS) operating at 1 kHz. This allows the push-button optimization of the wavelength. For example, in the case of BFO|SRO|STO the optimal wavelength was determined to be 1200 nm. In order to boost the signal intensity and ensure that data measurements are confined to single crystals, the OPA output is focused to a spot diameter of approx. 250 microns at the test film surface.
Another significant issue is to minimize the SHG signal that naturally occurs at the film/substrate interface. This signal is independent of film thickness and thus can be deconvoluted from the data.
Using this setup, the researchers then performed several different series of SHG experiments. In one type of experiment, they grew films of a pre-determined thickness (e.g., 20 unit cells) and then measured the polarization characteristics of the SHG signal. Specifically, they rotated the polarization of the linearly polarized OPA beam using a wave plate, while using a linear polarizer in front of the SHG photodetector (a photomultiplier). This early data showed pronounced anisotropy which, when correlated to the point group symmetry of the BFO, indicated that the film is naturally deposited with a domain pattern consisting of linear stripes. The team then applied an electric field ex situ to flip the ferroelectric polarization by 180° to confirm the ferroelectric nature of the thin layers.
This anisotropy analysis then allowed the team to further configure their ISHG setup for maximum sensitivity for BFO to perform film growth dynamic measurements. RHEED is measured simultaneously with ISHG to ensure the growth quality and to calibrate the ISHG yield to the thickness with unit cell (u.c.) accuracy. The data showed that the BFO growth rate in their PLD chamber was approximately one u.c. thickness in 20 seconds. With a SHG data accumulation time of 1 second, this results in 20 data points during each u.c. increase in film thickness.
The ETH group showed that SHG can be used to monitor the polarization in BFO films in situ. This data shows that polarization saturates at a thickness of approx. 12 unit cells. Standard RHEED was used to measure the film thickness during growth.
In their landmark paper (De Luca et al, Nature Comm. 8,149, 2017, DOI: 10.1038/s41467-017-01620-2) the ETH team published their first successful ISHG-PLD data. These showed that the ferroelectric SHG signal due to the BFO did not onset until a thickness of approximately 4 u.c.. This critical thickness value for spontaneous polarization is consistent with earlier literature data (not in situ) based on investigating SHG on static film samples. SHG polarization mapping of these films revealed a striped domain pattern. Furthermore, the bulk polarization value of the film is reached for a thickness as low as 10 u.c.
Defining and monitoring film polarization in heterostructures
Based on the successful demonstration of this ISHG-PLD technique, the team then began using this to investigate heterostructures and eventually model devices. The overarching purpose was to use the ISHG technique to monitor film polarization and depolarization effects in the technological relevant ultrathin regime and to refine methods for controlling polarization orientation. For example, it is known that chemical termination influences the polarization in thin layers, but what about the consequences of interlayer screening and coupling mechanisms in multilayers? For example, the investigators have already found out that in BFO|SRO bilayers the metal oxide SRO is an effective barrier to polarization coupling between the BFO layers. This offers the possibility of fabricating multilayer devices with single layer predefined up-down polarity.
Very recently, they have begun using ISHG to look at model prototypical devices, starting with a prototypical metal-ferroelectric-metal epitaxial system made of ferroelectric BaTiO3 and metal oxide SrRuO3. By tracking the polarization state in situ, they are bringing improved understanding of interface-related electrostatic effects in ferroelectric capacitors. For example, their latest ISHG data show that there is reduced conductivity and, hence, charge screening efficiency in the early growth stage of the SrRuO3 top electrode that promotes a breakdown of ferroelectric BaTiO3 into domains. Moreover, they have shown how a thermal annealing procedure can recover the single-domain state.
The Importance of amplifier stability
Traditionally, cutting edge lasers such as femtosecond amplifiers emphasized extreme performance with the understanding that this often meant trade-offs in both operational complexity as well as stability/reliability. However, Astrella is a modern one-box titanium:sapphire kilohertz amplifier designed from the ground up as part of Coherent’s ongoing program called the Industrial Revolution in Ultrafast Science. This program is based on the premise that Coherent’s scientific lasers could equal the reliability and ease of use of the company’s industrial lasers; the emphasis is on both 24/7 productivity and cutting-edge performance. A major contributor to the success of this program has been an investment in in-house HALT/HASS development and testing protocols. HALT stands for Highly Accelerated Life Testing where prototypes are deliberately and repeatedly pushed to failure to eliminate any design weaknesses. HASS means Highly Accelerate Stress Screening where production models are stressed to screen for any possible manufacturing errors – before shipping to the customer. This includes vibration and thermal stresses in a dedicated testing chamber. The laser output must still be in specification after this stressing program.
Trassin explains that there were two important productivity factors that cause them to select Astrella (plus TOPAS) – see the photo at top – as the ultrafast source for this research, “First the laser and OPAs are easy to control through a user-friendly software package. So we can focus on the ISHG experiments with very little attention to the laser source. And more important, Astrella is extremely stable with respect to extreme growth-laboratories conditions such as vibrations caused by vacuum pumps and temperature changes. Our chamber runs at up to 950° internally and heats the lab significantly during long experimental sequences. But the output of Astrella – power, beam shape and beam pointing are all unaffected by these ambient changes.”
Enabling heterojunction devices
In conclusion, a new optical diagnostic ISHG enables the polarization details of ferroelectrics oxide layers to be monitored in real time, as the layers are being deposited. This new capability may turn out to be an important tool for the microelectronics industry, enabling heterojunction devices with sophisticated functionality customized down to the atomic scale.
Written by Hans-Ulrich Emmerichs, Coherent