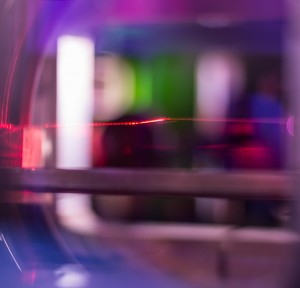
Researchers at the Pierre and Marie Curie University in Paris, France, have created a nano-mirror from only 2000 atoms of cesium. 200 times thinner than a human hair, the reportedly smallest mirror in the world offers the same level of reflectance as materials that require tens of millions of atoms. The breakthrough could represent a leap toward building light-based photonic circuits that will vastly increase computing speeds.
Bragg reflection
“The mirror works using the well-known phenomenon of Bragg reflection,” says Neil Corzo, postdoctoral fellow and lead author on the paper “Large Bragg Reflection from One-Dimensional Chains of Trapped Atoms Near a Nanoscale Waveguide.” published in Physical Review Letters. “In our case, we trap cold cesium atoms near a nanoscale waveguide — a one-dimensional nanofiber — where light can strongly interact with them. By engineering the distance between each atom, we can create conditions for a strong Bragg reflection, i.e., multiple constructive interference.”
The researcher explains that the atoms need to be separated by a specific distance, half of the wavelength of the light, which is 852 nm for cesium atoms. When the atoms are spaced precisely at half the wavelength, the light is making a round trip between two atoms — the distance is the wavelength and the interference can be constructive, based on the principle of Bragg Scattering.
Potential breakthrough for optical computing
The new nano-scale mirror, Corzo confirms, could represent a breakthrough for optical computing. The mirrors are also quantum mechanical objects: “You can play with them, you can flip the mirrors into a transparent state, for instance. Imagine a chain of different mirrors forming cavities. Some mirrors can be switched off, and information can be transported from one cavity to the others — a kind of quantum information bus configuration.”
The achievement is testament to the tremendous control Corzo and his collaborators were able to apply in the combination of cold atoms and nanoscale waveguides. “Ultra cold atoms are trapped close to a warm surfaces, and we can adjust the distance between them,” the researcher says. “They are localized with a better precision than 20 nm (trap depth).” Deeming this a “unique toolbox for further works,” Corzo says the most appealing application is to realize a switchable Bragg mirror.
Coupling cold atoms with light and controlling distance between them
“The novel development in our system is the ability to strongly couple cold atoms with light and the ability to design the distance between the atoms,” he notes, crediting the nanofiber for the feat. He explains that in previous experiments, the atoms were illuminated by optical beams in free space. Therefore, the photons interact weakly with each atom, warranting a large number of interaction. “Here, due to the coupling to the one-dimensional nanofiber, the interaction is much stronger,” Corzo emphazises. “Each atom can absorb a few percent of the incoming light.” This feature is what makes it possible to reduce the mirror from millions and millions of atoms to only a few thousands.
Two major challenges
On their path to a breakthrough in optical computing, however, the scientists had to overcome two major challenges: “First, the generation of an interface where to couple the cesium atoms with the nanofiber,” Corzo says. “The second challenge is to place the atoms at a correct distance from one another. This was solved via the use of a well-designed optical lattice (also inside the nanofiber), where we trapped the atoms at a distance of half the wavelength of the light being reflected, allowing a strong reflection.”
The technology’s future
Talking about the outlook for this technology, Corzo says, “The capability of coupling arrays of atoms to one dimensional waveguides is the key ingredient for freshly emerging proposals, including simulation by tunable interactions via the guided photons or quantum state engineering.” He also notes, however, that these protocols would require a much stronger coupling to the waveguide than what can be achieved with the nanofiber device he and his team have developed. “That being said, our paper shows in this context a cooperative phenomenon in a 1D configuration, which was to be demonstrated. It also shows the nice and versatile capability to control the positions of the atoms in an all-fibered setting.”
What motivated the research work
One proposal that motivated the work is the realization of integrated cavity-QED systems. Corzo envisions using such atomic mirrors, instead of real mirrors, and put an atom in the middle. The reference Cavity QED with atomic mirrors, referenced in the paper, can offer better scalability and enhanced coupling, according to the lead author.
Another motivation, underlined in the paper’s conclusion, is the ability to eventually control at the single photon level the reflexion (reflective/not reflective) of this device: “We can envision a single-photon switch that decides if another single photon is transmitted or reflected. That would be a unique device for quantum information processing, like a gate,” Corzo says. “To realize this feature and reach the single-photon level, the coupling also has to be improved further.”
Written by Sandra Henderson, Research Editor, Novus Light Technologies Today