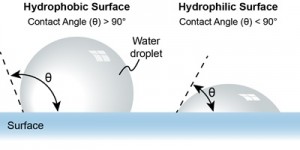
Water impacts nearly every aspect of human civilization and controlling its behavior is becoming an increasingly pressing scientific and engineering challenge. For example, while water covers 71% of Earth's surface, freshwater scarcity is a growing global issue, affecting over 700 million people worldwide. On the other hand, water and water vapor often shows up where it’s not wanted. This can range from floods to minor annoyances like a fogged-up car windshield or condensation inside an electronic device.
Scientists have long been aware that some surfaces found in nature have an ability to control water. For example, both the lotus leaf and rose petal strongly repel water, causing it to form surface droplets. Several plants and even some animals have surfaces or skins over which water readily spreads. The cornea of the human eye allows tears to spread evenly across its surface in order to maintain proper vision and protect it from debris.
Analysis of these surfaces has shown that their ability to control water is typically derived from two factors. The first is surface chemistry. This means the presence of surface molecules which have inherently water attracting (hydrophilic) or repelling (hydrophobic) characteristics based on their surface energy.
The second factor is surface shape (morphology). In particular, this involves the presence of very small-scale surface structures. Most significantly, the same structures will amplify whatever surface properties are already present, whether hydrophilic or hydrophobic.
The “contact angle” is the key parameter used for quantifying the wetting properties of a surface. This is defined as the angle between the tangent to a water droplet’s surface at the point of contact and the solid surface itself. A low contact angle (< 90°) means that the liquid readily spreads (hydrophilic). A contact angle above 90° indicates that liquid beads up, forming a droplet on the surface (hydrophobic). A contact angle of exactly 90°, associated with hemispherical droplets, indicates a neutral wetting state.
The most extreme states of water/surface interaction are called superhydrophobic and superhydrophilic. A superhydrophobic surface typically means a contact angle greater than 150°, while a superhydrophilic surface has a contact angle of less than 10°, often approaching 0°. Water on superhydrophilic surfaces spreads almost instantly, forming a thin film rather than discrete droplets.
Learning from Nature
A team of researchers at the Vorarlberg University of Applied Sciences (Dornbirn, Austria) has been working to understand and develop biomimetic surfaces (those that replicate nature’s designs) for water control. For example, the group analyzed the uniquely patterned microstructures found on the Namib Desert Beetle. These microstructures enable this beetle to collect moisture from fog, which is nearly the only reliable water source in its otherwise incredibly arid environment.
The beetle’s ability to “drink fog” derives from a combination of superhydrophilic and superhydrophobic areas on its elytra (hardened forewings). To employ these, the beetle tilts itself toward incoming fog, and water droplets form on its superhydrophilic bumps. The droplets continue to coalesce until they become so large that they are detached by gravity. Then, a series of superhydrophobic troughs funnel the water into the insect’s mouth.
The Vorarlberg researchers characterized the morphology and chemistry of the beetle’s elytra. They confirmed results that have been obtained previously by other researchers, namely that surface wettability is dramatically affected by the presence of “double hierarchical structures.” These are structures that contain features at two significantly different spatial frequencies, such as both microscale and nanoscale. The microscale features are responsible for directing water flow, while the nanoscale features enhance the surface energy. Together they increase the efficiency of capillary action and facilitate water transport across the surface.
The Vorarlberg group used a three-step laser-based fabrication method to mimic the pattern found on the Namib Desert Beetle artificially. In the first step, a Spectra‑Physics Spirit One femtosecond laser was used to create a double hierarchical pattern on the surface of Pyrex wafers. This transformed the already hydrophilic surface of the Pyrex into one which was superhydrophilic.
Next, a Teflon-like polymer was deposited on this surface. The addition of this low surface energy material on top of the laser-structured area switched it into one which was superhydrophobic.
Finally, the femtosecond laser was used again to remove the coating in only selected areas. This produced the desired pattern of alternately superhydrophilic and superhydrophobic areas.
The process for creating a pattern of superhydrophilic and superhydrophobic areas on a Pyrex wafer, and a photo of water droplets on the finished part.
Femtosecond Laser Patterning
There are diverse methods for producing so-called double hierarchical microstructures. These range from chemical etching to the deposition of nano-spheres onto microstructures. But femtosecond laser processing offers several key advantages over all other techniques, which is why it was chosen by the Vorarlberg team. For example, laser processing is maskless, which allows virtually any arbitrary pattern to be created without significant tooling or set up. This speeds up the research process and minimizes costs.
But, most importantly, femtosecond lasers are unique in their ability to create double hierarchical surface structures in a single step. Specifically, the high peak power levels of femtosecond lasers, which minimizes thermal diffusion and initiates nonlinear absorption effects (including, in some cases, multi-photon ionization), enables the formation of laser-induced periodic surface structures (LIPSS). LIPSS are periodic nanoscale features – usually smaller than the laser wavelength itself – that spontaneously appear during femtosecond laser ablation under certain conditions. LIPSS cannot be created using longer pulse width lasers.
The non-linear absorption induced by the high peak power femtosecond pulses also enables these lasers to ablate nearly any material. This includes transparent or brittle materials, like glass, as well as steel and silicon (although the exact morphology of the LIPSS features vary by material). This gives this technique broad applicability.
Ultrafast laser generated double hierarchical surface structure consisting of bumps that are about 15 µm wide and 10 µm high covered with LIPSS having a period of around 700 nm.
In this particular study, the research team first ablated grooves on the Pyrex with their Spectra‑Physics Spirit One configured for linearly polarized output at a wavelength of 520 nm, a pulse width of 380 fs, a repetition rate of 200 kHz, and a fluence of 6.2 J/cm². The focused spot size was 6 µm. Under these conditions, the laser was used to produce groove widths in the range of a few microns to tens of microns.
These operating conditions also enabled the laser to initiate the LIPSS phenomenon which automatically superimposed smaller periodic features on the grooves. These had a typical size and period in the sub-micron range.
In the final step, the same Spectra-Physics Spirit One laser was operated at a much lower fluence of 2.1 J/cm². This allowed removal of just the thin Teflon layer without affecting the underlying microstructures created previously.
Reigning in Water
Subsequently, a team at MKS’ Spectra-Physics continued these investigations in collaboration with the group at Vorarlberg University. Based on the success of their experiments, they filed for a joint patent on their method for the fast and flexible fabrication of superhydrophilic micropatterns on a superhydrophobic background. The technique is called ClearSurface.
One obvious use for ClearSurface is microfluidics. In this field, controlling the flow of minute amounts of fluids is crucial for applications such as lab-on-a-chip devices, cell culturing, drug delivery systems, and many more.
Using ClearSurface, intricate microchannels can be structured to direct liquid flow precisely where it is needed. Due to the high wettability gradient created by the pattern, surface tension alone confines water within the predefined superhydrophilic microchannels and reservoirs. The water moves through these without the need for pumps or external forces. The laser-based ClearSurface process is highly flexible, and could be applied to other fluids, as well.
In ClearSurface, glass is microstructured using a femtosecond laser (top). A closeup shows the edge of the patterned region (middle). When wetted, water automatically spreads over the entire pattern and is contained within it solely by surface tension (bottom).
A less exotic application for ClearSurface is anti-fogging technology for products like eyeglasses, car mirrors, helmet visors, and windows. Traditional anti-fog coatings make surfaces more hydrophilic to reduce droplet formation. But a laser-fabricated superhydrophilic surface would force water to spread more evenly across it instead of forming droplets at all. It could then employ superhydrophobic zones to efficiently carry water away entirely.
View through fogged glass, and glass processed with ClearSurface exposed to the same conditions of temperature and humidity.
In The Rime of the Ancient Mariner, poet Samuel Taylor Coleridge laments “Water, water, every where, Nor any drop to drink.” This summarizes the fact that water is all around us yet doesn’t always take the form that we would like. By employing femtosecond laser processing, we come a step closer to controlling this essential and ubiquitous substance.
Written by Victor Matylitsky, Ph.D., Senior Product Marketing Manager, Spectra-Physics Products, MKS Instrument, ,