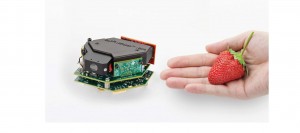
Spectroscopy has come a long way since its inception as lab-based technology. Handheld near-infrared (NIR) spectrometers continue to shrink in size and cost thanks in part to the advent of novel system architectures that leverage micro-electro-mechanical systems (MEMS) components. Let's explore how these hardware optimizations can enable a simpler and more portable future for the spectroscopy industry.
NIR spectroscopy
Spectroscopy serves as a powerful tool for identifying samples based on their response to a broad range of wavelengths. Specifically, NIR spectroscopy excites the sample with wavelengths of light typically spanning 780-2500nm. Depending on the physical state of the sample material, the spectral response can be gauged using reflectance measurements (solids) or absorbance measurements (liquids and gases).
Spectral signatures in the 780-2500nm region are dominated by the presence of hydrogen bonds such as O-H, C-H, N-H and S-H. In this way, the NIR waveband is particularly well-suited for food and agricultural monitoring, health diagnostics, petrochemical processing and pharmaceutical manufacturing. Within the NIR waveband, each spectroscopy application has unique requirements for wavelength range and chemometric analysis. For example, a 900-1700nm instrument can provide information regarding water (H20) and sucrose (C12H24O12) content [1]. Extending the wavelength range of the instrument to 2500nm allows for discovery of additional organic compound signatures and can improve results in pharmaceutical process monitoring [2].
The total bill of materials (BOM) cost of the instrument can be impacted by the chosen wavelength range. A short-wave NIR system could leverage an inexpensive silicon detector for measurements up to 1050nm. After 1050nm, a more expensive Indium Gallium Arsenide (InGaAs) detector is often required. Past 1700nm, the InGaAs material normally requires cooling, especially with multi-pixel linear array detectors, in order to maintain performance requirements. Because of the more expensive InGaAs substrate and additional cooling elements, InGaAs linear array technology can be preventatively expensive for low-cost handheld instruments.
Innovations in spectrometer architecture
Given the cost challenges of traditional dispersive spectroscopy with an InGaAs array detector, much NIR spectrometer innovation has centered on reducing the number of system components. Replacing the dispersive grating relay with a linear variable filter (LVF) is one such example. The LVF architecture reduces light throughput but also greatly shrinks the spectrometer footprint by removing the path from the grating to detector. Other innovative optical designs employ a transmissive grating architecture, which streamlines the system footprint while minimizing light loss. Another architecture uses a scanning grating to relay light directly to a single-point detector, eliminating the need for the aforementioned multi-pixel InGaAs array. Single-point detectors have significant advantages over array detectors in cost, size and performance.
The adoption of MEMS technology within spectrometer architectures, along with the use of single-point detectors, builds on the theme of cost reduction and portability. The integration of robust MEMS components into a spectrometer light path can both shrink the instrument footprint and add new performance capabilities. Key careabouts when selecting MEMS components include performance reliability and high-volume manufacturing stability.
Examples of proven MEMS technology are the DLP® NIR chipsets from Texas Instruments. This technology provides high-fidelity light modulation for compact, programmable and high-performance spectrometers. Specifically, the TI DLP2010NIR and DLP4500NIR enable exciting new wavelength control features such as Hadamard patterns and slew scan dynamic programmability. Other emerging MEMS technologies, including the Fabry-Perot interferometer and Michelson interferometer, show promise for simplifying instrument architecture but may still face challenges in meeting lab performance requirements for signal-to-noise ratio and resolution metrics.
While there are many spectrometer architecture options, MEMS technology proves increasingly attractive. Dynamic programmability, cost reduction, use of single point detector and elimination of large moving pieces are a few benefits that a MEMS-based architecture can offer. These benefits, in combination with reliable system integration, become even more critical during field deployment.
Mobile applications and industry outlook
Compact, high-performance NIR spectroscopy instruments have contributed to the emergence of field applications where in-situ measurements could add value for both individual users and industrial companies. Linking these spectrometers to cloud databases over Wi-Fi or Bluetooth® wireless connectivity via a mobile device introduces full predictive capabilities of the lab at the sample. In this way, integrated spectrometers can serve as high-performance optical sensors on the edge of the network. When spectrometer hardware facilitates this high-fidelity data aggregation in the cloud, the Internet of Things (IoT) can dynamically improve process efficiency. Pioneering applications for IoT mobile sensing include food safety, remote agricultural monitoring and process monitoring for pharmaceutical manufacturing.
Perhaps the most exciting NIR spectroscopy industry trend is the open-source model. Cutting-edge technology developers such as Texas Instruments, Consumer Physics, and Si-ware have released various Software Development Kits (SDKs) to encourage innovation. An example of a company pioneering low-cost NIR spectrometer architecture for industrial IoT applications is KS Technologies. In addition to offering free iOS and Android applications and SDKs for the DLP NIRscan Nano evaluation module seen in Figure 1, the company has applied their expertise in mobile data systems and IoT infrastructure to the emerging mobile NIR sensing market.
Due to the low-cost and open-source nature of these platforms, the offerings lend themselves to collaboration with university chemometrics experts to augment applications knowledge. In this way, accessible hardware coupled with open-source software facilitates algorithm and chemometrics development, which in turn fuels the NIR spectroscopy ecosystem. Future industry growth and innovation will depend on continued collaboration between experts.
Given the powerful capabilities of NIR spectroscopy analysis, much of the industry focus has centered on transferring this high-performance analysis from the laboratory to the field. The resulting industry breakthroughs in NIR spectrometer architectures are fueling a new wave of innovative, mobile measurement capability. This innovation is consistent with the mobile trend of the 21st century and has a logical intersection point with the IoT revolution. As spectroscopy evolves from an expensive lab-only operation to producing precise data from the palm of your hand, what will you measure?
[1] B.M. Nicola¨ı et al. / Postharvest Biology and Technology 46 (2007). 99–118.
[2] Chang, Cheng-Wen, "Near-infrared reflectance spectroscopic measurement of soil properties" (2000). Retrospective Theses and Dissertations. Paper 12315.
Written by Dorsey Standish, Texas Instruments DLP Products advanced light control program manager.