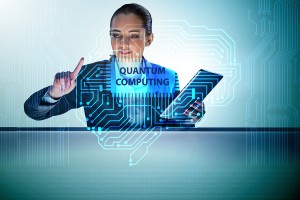
Quantum computers promise to transform information technology. Perhaps only a few years from now, they will be performing tasks impossible for any classical machine, powering data science, engineering and drug design. The commercial quantum computers of the future are very likely to require very precise control of light, creating a multi-billion-dollar market for lasers and other photonic components.
This new kind of computing uses a phenomenon known as superposition, in which a quantum system can adopt more than one state at a time. An atom, for example, can be partly in a high energy state, partly low, with the proportions of the two states giving the probability of seeing each outcome when you make a measurement. Such a two-state quantum system is known as a qubit, the quantum equivalent of a classical bit of information. In a digital quantum computer, qubits are set up to encode a calculation, then made to interact with one another through quantum logic gates, and finally their states are measured to give the answer.
For certain tasks, the power of quantum computers grows exponentially with size, inevitably overtaking computers based on classical bits of information
In principle a quantum computer can make some types of calculation far faster than any classical machine. For example, they can rapidly calculate the prime factors of large numbers. This is a concern because a widespread form of data encryption known as RSA relies on the mathematical difficulty of factorisation, and it will be undermined when quantum computers grow powerful enough. Another quantum algorithm provides a way to rapidly search an unordered database, and this could have a much more positive impact. The total amount of data being generated around the world is growing even faster than classical computing power, and quantum computing may eventually be the only way to analyse this this global information explosion.
Quantum computers are also expected to be supreme in a wide range of optimisation problems in engineering and elsewhere; and they will be unparalleled at simulating molecules and other quantum systems, becoming powerful tools for designing drugs and advanced materials.
Such rich promise means that many companies and academic groups are trying to develop a large-scale practical quantum computer. The challenge is that quantum states are inherently delicate. Random environmental effects rapidly wipe their information content, so qubits have to be isolated, often with the help of very low temperatures and high vacuum.
Google, IBM, and Rigetti use qubits based on superconducting circuits chilled to below 0.1 Kelvin in a device known as a dilution refrigerator. They have achieved demonstrator machines with tens of qubits, but this approach may not scale up well. Superconducting logic gates have shown relatively low fidelity, meaning high error rates (see figure below). While some level of error can be corrected, the correction sucks up enormous amounts of processing power, requiring many physical qubits to implement one logical qubit. And squeezing a much larger number of qubits into one refrigerator is likely to become increasingly difficult – especially while keeping the fidelity high.
Many other approaches are being pursued, including qubits based on semiconductors, quantum dots, fullerenes and molecules in solution. Several rely on precise control of light, including one approach in which photons themselves form the qubits; but perhaps the most promising approaches use light to manipulate individual atoms and ions, where energy levels serve as the qubits.
The role of lasers
Lasers play many roles in these machines. They cool atoms down to a very low temperature and then trap them in an optical lattice – an interference pattern forming a periodic array of potential energy minima. Ions can also be held in arrays and shuttled around using laser fields. Some versions have optical qubits, with lasers tuned to the relevant energy levels in order to set up, link and measure the qubits; others use microwaves to control qubits stored in much lower-energy transitions. Photodetectors, optical modulators and other photonic devices are also essential, along with control electronics.
Ion and atom systems have the most reliable quantum logic gates yet achieved. For example, a team led by David Lucas at the University of Oxford, UK, has demonstrated a two-qubit microwave gate with a fidelity of 99.9%, meaning an error rate of only one in a thousand. Such precise control is easier in processors with only a handful of qubits, so the Oxford group’s aim is to build many such small processors and then use laser light to shuttle quantum information between them, forming one larger machine.
Laser-based quantum computers are setting the pace, with ion logic gates showing the best fidelity and neutral atoms among the largest by qubit count
Optical technologies can enable a powerful quantum computer, with hundreds of qubits and low error rates. These machines will need more powerful lasers so the light can be distributed to more and more nodes; and each approach has particular needs. Microwave qubits need very low phase noise. Optical ion qubits need ultra-narrow linewidth, down to the Hz level. Neutral atoms need lasers with precise power stability, or else the optical lattice shakes and rapidly degrades the fragile quantum information within. Cold atom approaches will also require new optical switching technology to address different qubits. At the same time, the hardware needs to become more rugged and easier to use than existing fiddly experimental setups, and it must be more compact, with photonic components integrated onto single chips.
Photonic opportunities
To meet all these challenges, photonics-based computers can exploit developments in other fields of quantum technology. Cold-atom quantum sensors for magnetism, gravity and acceleration are close to commercialisation, and optical quantum clocks are already there, thanks to new generations of solid-state lasers and other optical components. The overall quantum technology market for lasers grew from $27 million in 2015 to $87 million in 2018, and is projected by IQT Research and Tractica to reach well over a billion by 2024.
Quantum computing is a relatively small part of that today, but it promises to become a huge industry even if these machines are unlikely to become mass-marked devices in the next couple of decades. One recent report from Boston Consulting predicting a global applications market of up to $60 billion in 2035, and between $260 and $295 billion by 2050. There’s a clear business opportunity for whoever can provide the tools to make this happen – and chances are that the picks and shovels of this quantum goldrush will be lasers and photodetectors.
Written by Dr Graeme Malcolm OBE, CEO and Founder, and Dr Nils Hempler, Head of Innovation, M Squared Lasers